Logic Gates: Building Blocks, Types, and Applications in Digital Systems
Logic gates, such as AND, OR, and NOT, are the essential building blocks of combinational logic circ…….
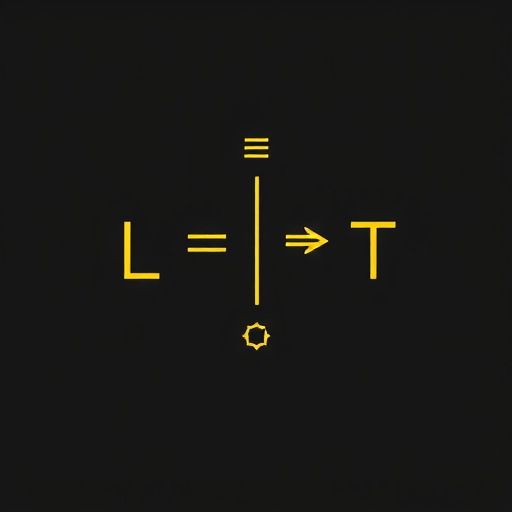
Logic gates, such as AND, OR, and NOT, are the essential building blocks of combinational logic circuits, which process binary data for various digital tasks. These gates form the foundation for complex circuit design, enabling operations from simple calculations to advanced data processing. While they offer speed and efficiency, scalability and lack of memory capability pose challenges. Strategic placement and precise wiring of these fundamental components are crucial for creating effective digital systems that power modern technology.
Logic gates, the fundamental building blocks of digital systems, form the backbone of combinational logic circuits. This article delves into the intricate world of these circuits, exploring how logic gates combine to process and manipulate data. We’ll dissect various gate types, dissect design principles, uncover applications from microprocessors to memory systems, and analyze the circuit’s advantages and limitations in today’s digital landscape.
Understanding logic gates is key to navigating the complexities of combinational logic.
- Understanding Logic Gates: The Building Blocks
- Types of Combinational Logic Gates
- Design and Construction of Circuitry
- Applications in Digital Systems
- Advantages and Limitations: A Balanced Perspective
Understanding Logic Gates: The Building Blocks
Logic gates are the fundamental building blocks of combinational logic circuits, acting as the essential components that process and manipulate binary data. These simple yet powerful elements form the backbone of digital systems, enabling complex operations through their ability to perform basic logical functions. AND, OR, NOT—these are the primary logic gates, each with its unique behavior and application.
Understanding how these gates operate is crucial when designing or troubleshooting combinational circuits. By combining and arranging logic gates, engineers can create intricate circuits capable of performing a wide range of tasks, from simple calculations to advanced data processing. The versatility and efficiency of logic gates make them an indispensable tool in the world of digital electronics.
Types of Combinational Logic Gates
Combinational logic circuits, as the name suggests, rely on the combination or arrangement of various logic gates to perform specific computational tasks. These gates are fundamental building blocks that process and manipulate binary data by applying logical operations. There are multiple types of combinational logic gates, each designed for distinct functions, enabling efficient and effective circuit design.
The AND gate is a basic combinational logic gate that performs the bitwise conjunction operation, returning a 1 only if both inputs are 1. OR gates, on the other hand, produce a 1 when at least one input is 1, making them essential for tasks requiring majority voting or simple decision-making processes. NOT gates, also known as inverters, reverse the state of their input, from 1 to 0 or vice versa, providing a simple yet crucial function in circuit logic. Additionally, more complex gates like NAND (NOT AND), NOR (NOT OR), XOR (exclusive OR), and XNOR (exclusive NOR) offer advanced logical operations, expanding the capabilities of combinational circuits for intricate calculations.
Design and Construction of Circuitry
The design and construction of combinational logic circuits rely heavily on the strategic arrangement of logic gates, which serve as the building blocks for complex computational functions. Engineers utilize these gates, such as AND, OR, NOT, NAND, NOR, and XOR, to create intricate digital systems that process inputs according to specific Boolean functions. By combining these gates through careful wiring, designers can build circuits capable of performing a wide range of operations, from simple data manipulation to advanced arithmetic calculations.
The construction process involves meticulous planning to ensure the circuit’s functionality and efficiency. Each logic gate is placed strategically based on its logical operation and output requirements. Connections between gates are established using conductive pathways, typically implemented with wires or trace routes on integrated circuits (ICs). Proper routing of these connections is crucial for maintaining signal integrity and minimizing delays, ensuring that the circuit operates as intended.
Applications in Digital Systems
Combinational logic circuits, built upon interconnected logic gates, form the backbone of modern digital systems. These versatile circuits process and manipulate binary data through a series of logical operations, making them indispensable in various applications. From simple arithmetic calculations to complex data processing tasks, logic gates enable efficient and accurate information handling.
In digital systems, combinational logic is employed for tasks such as data encoding, decoding, filtering, and sorting. For instance, in microprocessors, logic gates facilitate the execution of instructions by performing operations on registers and memory addresses. Additionally, these circuits power up various peripherals like keyboards, mice, and displays, ensuring seamless interaction with users. The ability to create intricate logic combinations through gate interconnexions allows for the development of sophisticated digital devices that underpin our modern technological landscape.
Advantages and Limitations: A Balanced Perspective
Combinational logic circuits, built from interconnected logic gates, offer both significant advantages and limitations that must be carefully considered in circuit design. Among their key benefits is speed and efficiency; these circuits process inputs immediately, producing outputs without storing intermediate results, making them ideal for time-sensitive applications. Additionally, combinational logic is inherently simple to implement and debug due to its direct input-output relationships, streamlining the design and testing phases.
However, limitations exist, particularly in terms of scalability and complexity. As circuits grow larger, managing interconnections between gates can become intricate, leading to potential timing issues and increased power consumption. Furthermore, combinational logic lacks memory, restricting its ability to perform operations that require data retention. These constraints necessitate careful design choices, especially for complex systems, where balancing speed with manageability is crucial to achieving optimal circuit performance.